Vitamin E is an important fat-soluble vitamin, composed of tocopherol and tocotrienol, which has various physiological functions such as antioxidant, anti-inflammatory and lipid regulation, and has been widely used in food industry, cosmetics, dietary supplements, pharmaceuticals and animal nutrition.
At present, the production of vitamin E mainly relies on plant extraction and chemical synthesis, and the development of synthetic biology and biotechnology tools has opened up new ways to produce natural vitamin E.
Ye Lidan’s team at Zhejiang University published a review in the Journal of Agricultural and Food Chemistry. Evolution of Vitamin E Production: From Chemical Synthesis and Plant Extraction to Microbial Cell Factories, from chemical synthesis and plant extraction to microbial cell factories, from chemical synthesis and plant extraction to microbial cell factories, It provides reference for promoting sustainable green production of vitamin E.
Extracted from natural resources
Natural vitamin E is mainly concentrated in vegetable oils and is usually extracted from the deodorizing distillation residue of vegetable oils and oilseed crops.
Several methods have been developed to extract natural vitamin E, including saponification extraction, solvent extraction and supercritical fluid extraction.
A common extraction method involves esterifying the fatty acids in the deodorized distillation residue with lower alcohols, and then crystallizing by neutralization and cooling to obtain a concentrated natural vitamin E product while maintaining its biological activity.
Plant-based extraction methods face challenges such as high costs, climate dependence, complex purification processes and potential impacts on biodiversity, which make it difficult for plant-based extraction methods to consistently meet market demand.
Chemical synthesis of alpha-tocopherol
Chemically synthesized vitamin E accounts for more than 80% of global vitamin E production and is mainly used in animal feed.
In 1938, the first successful chemical synthesis of alpha-tocopherol was made using a “one-step condensation” method, which involved the condensation of 2,3, 5-trimethylhydroquinone (TMHQ) as the main ring structure with isophytol (IP) as the side chain under acid catalysis.
This method has been improved over the years and the current yield is over 95%.
To meet the growing demand for vitamin E, researchers have developed and optimized various methods for synthesizing its precursors.
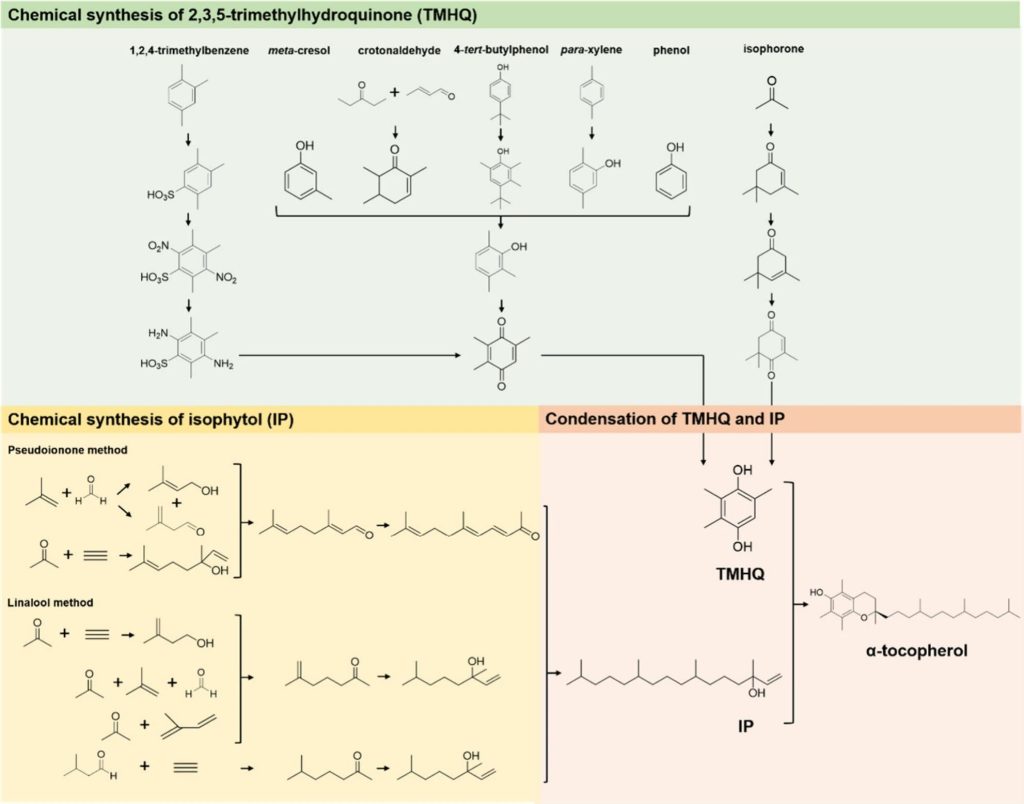
The industrial synthesis route of TMHQ can be divided into several different methods, using p-xylene, crotonaldehyde, 4-tert-butylphenol, cresol, isophorone, phenol and 1,2, 4-trimethylbenzene as starting materials.
The preparation of IP follows two main routes, based on key intermediates:
Pseudo-ionic ketone method and lavender alcohol method.
Although the chemical synthesis of vitamin E offers significant advantages and has an important place in the feedstock industry, the synthesis of IP remains a challenge.
The process requires seven catalytic steps, including a multi-step alkyne reaction under high pressure, which requires complex equipment and strict safety measures.
Biofarnesene as an intermediate semi-synthesis of alpha-tocopherol
In 2014, the research team of Liu Tiangang, School of Pharmacy, Wuhan University, innovated and proposed a semi-synthetic vitamin E production method combining biosynthesis and chemical synthesis, which used microbial fermentation to produce farnesene as an intermediate for the synthesis of IP, and then used for the synthesis of alpha-tocopherol.
The researchers engineered a key enzyme in E. coli to create a strain capable of efficiently synthesizing farnesene.
Through target engineering, farnesene production can systematically increased to a shaker scale of 1.1g/L.
Based on this, in 2022, the team adopted Saccharomyces cerevisiae as the host organism and significantly increased the production of farnesene to 55.4g/L through protein engineering and systematic metabolic engineering strategies.
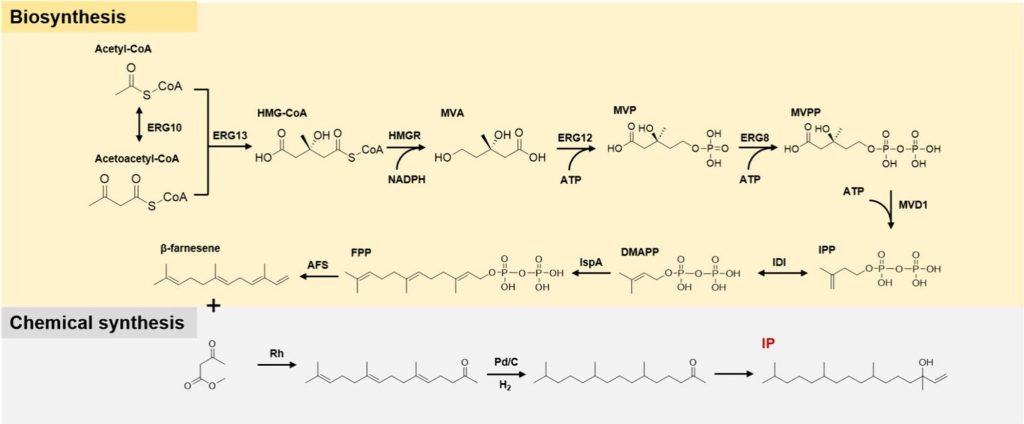
Using biosynthesized farnesene as a precursor, the synthesis of phytoalcohols can completed in just three steps, reducing the need for potentially hazardous materials.
It is important to note that the final stage of this vitamin E synthesis method still relies on chemical methods.
A limitation of this semi-synthetic route, similar to conventional chemical synthesis using TMHQ and IP, is that it is not possible to produce stereochemically pure compounds, but instead produces racemic mixtures of alpha-tocopherol, which may be less biologically active than the natural form.
Research progress of vitamin E biosynthesis
Although methods for chemical synthesis and semi-synthesis of alpha-tocopherols have established, these methods fall short in producing the specific stereoisomers of vitamin E that are essential for its biological activity.
Due to concerns about chemical residues, the application of synthetic vitamin E mainly limited to animal feed additives.
There is a growing preference for naturally synthetic vitamin E in the health, pharmaceutical and cosmetic industries.
In various forms, tocotrienol has received significant attention due to its superior antioxidant and anti-inflammatory properties over tocopherol.
To meet the growing demand, research is increasingly focusing on converting model microbes into vitamin E cell factories through plant genetic improvement or through synthetic biology methods to increase the production of natural biosynthesis of vitamin E.
Biosynthetic pathways of vitamin E
The synthesis pathway of vitamin E in Arabidines and algae has long elucidated, and this pathway highly conserved between algae and plants, requiring two key precursors: homovanillic acid (HGA) and geranylgeranylpyrophosphate (GGPP).
HGA produced through the shikimic acid pathway, which involves several steps, including condensation of PEP and E4P to shikimic acid, conversion of shikimic acid to prebenzoic acid, then conversion to tyrosine, 4-HPP, and finally oxidation of 4-HPP to HGA catalyzed by 4-hydroxyphenylpyruvate dioxygenase (HPPD).
GGPP synthesized mainly through the MEP pathway in plastid, which begins with the condensation of G3P and pyruvate, catalyzed by DXP synthase (DXS) to produce 1-deoxy-D-xylulose 5-phosphate (DXP), which, after several steps, forms IPP and DMAPP, and then condensates to form GGPP.
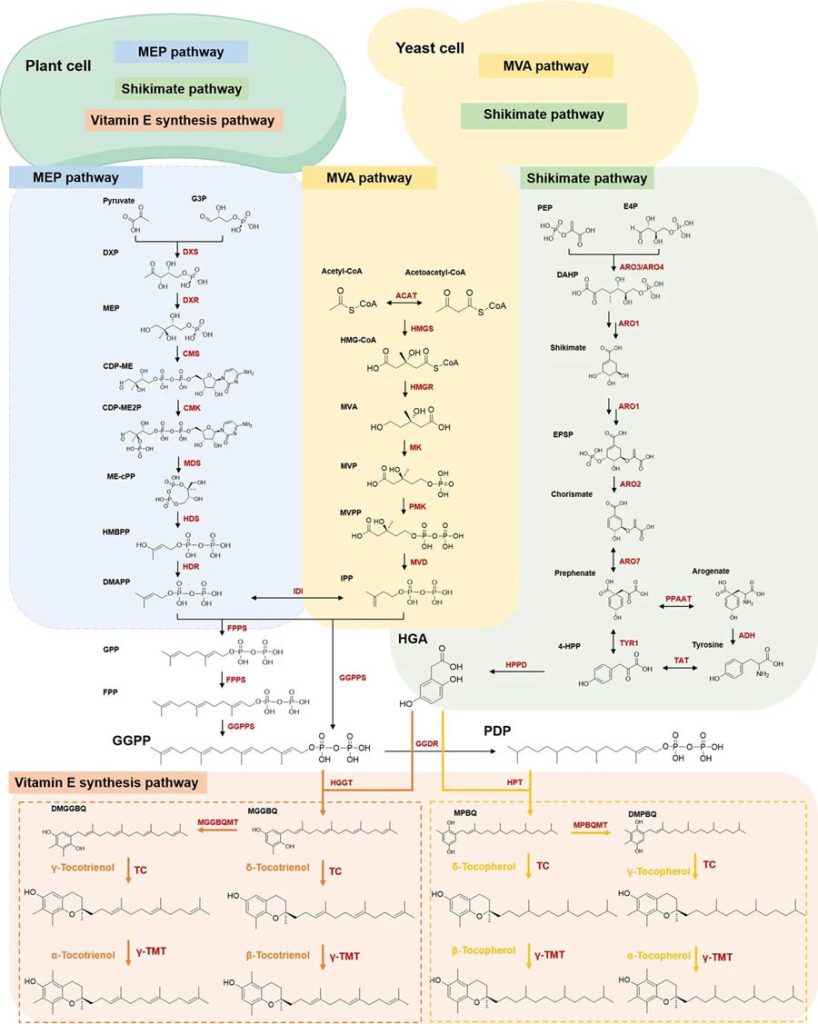
For tocotrienol, the condensation reaction of HGA and GGPP catalyzed by geranyltransferase (HGGT) to form 2-methyl-6-geranylbenzoquinolinol (MGGBQ).
For tocopherols, GGPP is first reduced by geranylgeranyldiphosphate reductase (GGDR) to phytol diphosphate (PDP), which then condensed with HGA and catalyzed by homovanilate phytol transferase (HPT) to form 2-methyl-6-phytol benzoquinolinol (MPBQ).
These intermediates (MGGBQ and MPBQ) can further converted by methyltransferase to 2, 3-dimethyl-5-geranyl benzoquinolines (DMGGBQ) and 2, 3-dimethyl-5-phytol benzoquinolines (DMPBQ), which then catalyzed by tocopherol cyclase (TC) to produce tocotrienols and tocopherols in the δ and γ forms.
Gamma-tocopherol methyltransferase (gamma-TMT) converts these forms into their respective β and α isomers.
Metabolic engineering to increase plant vitamin E production
After elucidating the genes for vitamin E biosynthesis, the study employed a variety of strategies to increase vitamin E levels in plants.
One common approach is to enhance the supply of key intermediates such as HGA and GGPP or PDP through metabolic engineering, for example overexpression of yeast source PDH in plants has shown to increase the supply of 4-HPP, thereby enhancing the production of HGA and its derived compounds;
By removing the feedback inhibition of tyrosine on ARO3/ARO4 activity in shikimic acid pathway, the supply of shikimic acid can increased.
Adjusting the ratio between PEP and E4P or optimizing their synthetic pathway can also enhance the carbon flux in the shikic acid pathway.
Overexpression of genes directly involved in vitamin E synthesis has also shown to be effective, for example, increasing HGGT expression in soybean and corn seeds significantly increased vitamin E content, and total tocotrienol levels in soybean seeds increased by up to 15 times compared to wild-type varieties;
The comprehensive approach also showed promise.
By combining RNai-mediated inhibition of chlorophyll synthase with overexpression of HPT and TyrC, the tocopherol content in Arabidopsis seeds increased by nearly three times, while the tocopherol slightly accumulated.
Overexpression of HPPD has shown to increase the ratio of tocotrienol to tocopherol.
Development of a microbial cell factory for vitamin E biosynthesis
Advances in computer-aided modeling, systems biology, synthetic biology, and protein engineering have made it possible to design and construct synthetic pathways for complex plant natural products, including the biosynthesis of tocotrienol in microbial cell factories.
Delta-tocotrienol synthesis in E. coli
The allosynthesis of δ-tocotrienol achieved for the first time by overexpressing PpHPPD, PaCrtE, SyHPT and AtVTE1 in Escherichia coli with a yield of 15μg/g DCW.
Subsequent studies solved the metabolic burden caused by multiple copy plasmids by integrating these genes into the E. coli genome, and further enhanced the MEP pathway through genomic integration IDI and DXS, increasing the accumulation of MGGBQ (a precursor of tocotrienol) to 1425μg/g DCW.
Synthesis of tocotrienol in Saccharomyces cerevisiae
Saccharomyces cerevisiae is the first choice for the production of high value natural products of plant origin due to its ability to express complex proteins, perform post-translational modifications, and adapt to industrial conditions.
By introducing and overexpressing exogenous HPPD, HGGT, TC, MPBQ-MT and γ-TMT enzymes, combined with endogenous shikimic acid pathway and MVA pathway, tocotrienol has successfully synthesized in Saccharsaccharus cerevissae.
By integrating PpHPPD, SyHGT and AtVTE1, and overexpressing tHMG1 and GGPPSsa to enhance GGPP supply, the yeast strain producing 4.10 mg/L delta-tocotrienol successfully constructed.
In the same year, the team developed a yeast strain capable of producing all four tocotrienols, and by integrating codon-optimized genes, the total tocotrienol production reached 313μg/g DCW in shake flask culture;
By enhancing precursor supply and overexpression of key enzymes, total tocotrienol production could reach 2.6 mg/g DCW.
Through temperature control and dynamic expression regulation of synthetic pathway genes, high density fermentation of tocotrienol achieved with a yield of up to 320 mg/L, the highest yield reported so far.
The demand for vitamin E is growing because of its health benefits.
While traditional production relies on chemical synthesis and plant extraction, emerging methods include semi-synthesis and de novo biosynthesis.
Natural extraction has high biological activity but low yield, chemical synthesis has low cost but large environmental pollution, and semi-synthesis and de novo biosynthesis are more sustainable.
The production of tocotrienol in saccharomyces cerevisiae has achieved through metabolic engineering, but the yield is not up to the industrial standard.
Source of information:
https://doi.org/10.1021/acs.jafc.4c08813