Chinese scholars at the University of Oxford have published the results of PNAS, engineering bacteria to build efficient hydrogen energy bionanometer reactor, opening a new era of sustainable energy
Under the dual challenges of global energy crisis and climate change, finding efficient and sustainable clean energy has become a common goal of the scientific community.
Hydrogen is regarded as the most potential future energy source due to its high energy density and environmental protection properties because the combustion product is only water.
However, the current hydrogen production methods still face many bottlenecks, the traditional water electrolysis hydrogen production relies on expensive precious metal catalysts, and high energy consumption;
Although the cost of microbial electrochemical system is low, there are problems of low hydrogen production and efficiency.
Based on this, Professor Huang Wei’s team at the University of Oxford has proposed a new hydrogen production strategy through innovative combination of nanomaterials engineering and synthetic biology – the construction of a “biofilm reactor” in the periplasma space of bacteria Shewanella oneidensis MR-1.
This design takes full advantage of the electroactive and programmable properties of the bacteria to concentrate foreign electrons, protons and catalytic enzymes in the nanoscale reaction space, which greatly improves the efficiency of hydrogen production.
More importantly, this system uses light and electricity as the driving force to achieve clean and efficient hydrogen production, opening up a new direction for future green energy technologies.
The work published in PNAS under the title “Engineering bionanoreactor in bacteria for efficient hydrogen production.”
Shewanella oneidensis MR-1 acts as an “electroactive” bacterium capable of efficiently transporting electrons and protons in the periplasmic space (about 20-30 nm wide) between its inner and outer membranes.
Using the reducing power of Shewanella oneidensis MR-1, the research team successfully self-assembled iron sulfide (FeS) nanoparticles in the bacterial periplasmic space.
The functional test showed that the electron transfer rate of bacteria carrying FeS nanoparticles on the electrode significantly improved.
In the electrochemical test, the modified bacteria obtained electrons from the electrode more efficiently, providing favorable conditions for hydrogenase reaction.
The current density of the modified strain reached -37 μA/cm², more than twice that of the unmodified strain, which confirmed that the FeS nanoparticles significantly improved the electron transport capacity of the bacteria and provided a possibility for improving the hydrogen production efficiency.
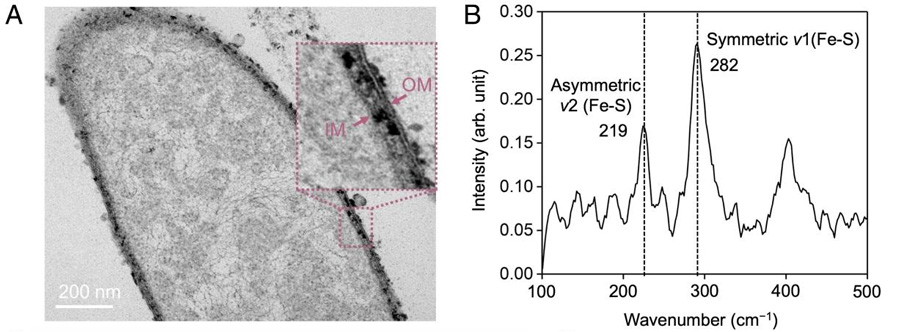
To enhance electron transfer efficiency, the research team used the natural reducing ability of Shewanella oneidensis MR-1 to reduce graphene oxide (GO) to reduced graphene oxide (rGO), which coated on the electrode surface to form a highly conductive coating.
Electrochemical tests showed that rGO significantly improved the electron transfer efficiency between the bacteria and the electrode, and increased the hydrogen generation rate from 7.82 μmol/mg/d to 25.69 μmol/mg/d.
When combined with FeS nanoparticles, hydrogen production further increased to 43.11 μmol/mg/d, which was 5 times that of the unmodified system.
In order to solve the problem of insufficient proton concentration during hydrogen generation, researchers designed a light-driven proton pump Gloeobacter rhodopsin (GR) on the inner membrane of bacteria.
GR is a light-sensitive protein of rhodopsin class, which can efficiently pump protons into periplasmic space under light conditions.
In order to enhance the absorption efficiency of light energy, GR further combined with the pigment molecule canthaxanthin (CAN), which able to capture a wider range of light waves and transfer light energy to GR, thus maximizing the use of sunlight to drive proton transfer.
Experiments show that the proton concentration of the system significantly increased under light, and the current density of the system increased from -58 μA/cm² in dark environment to -64 μA/cm², which provides an ideal chemical environment for hydrogenase, and the hydrogen yield increased by 35.6%.
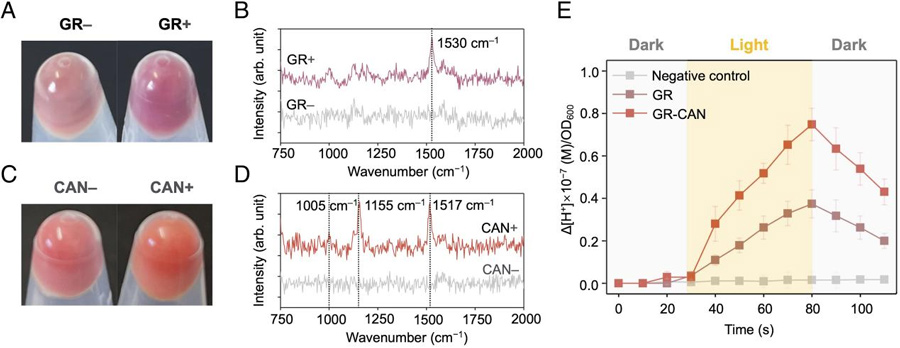
In order to further improve the efficiency of hydrogen production, the research team genetically engineered Shewanella oneidensis MR-1 to overexpress the enzyme that catalyzes hydrogen production – [FeFe] -hydrogenase, significantly enhancing the proton and electron binding efficiency.
The results showed that the hydrogen generation rate of the modified strain reached 80.4 μmol/mg/d, which 10 times that of the wild-type strain, and the Faraday efficiency of the system also increased to 80%, demonstrating the remarkable optimization of the catalytic reaction in periplasmic space.
By integrating FeS nanoparticles, RGO-modified electrodes, light-driven proton pump systems, and hydrogenase overexpression technologies, the team’s bionanoreactor has demonstrated unprecedented performance advantages in photoelectrochemical systems.
When a voltage of -0.75V is applied and operated under light conditions, the hydrogen generation rate of the system reaches 80.4 μmol/mg/d.
If the voltage is further increased to -0.85 V, the hydrogen production can be increased to 123.55 μmol/mg/d.
This research has led to breakthroughs in several key aspects of hydrogen production.
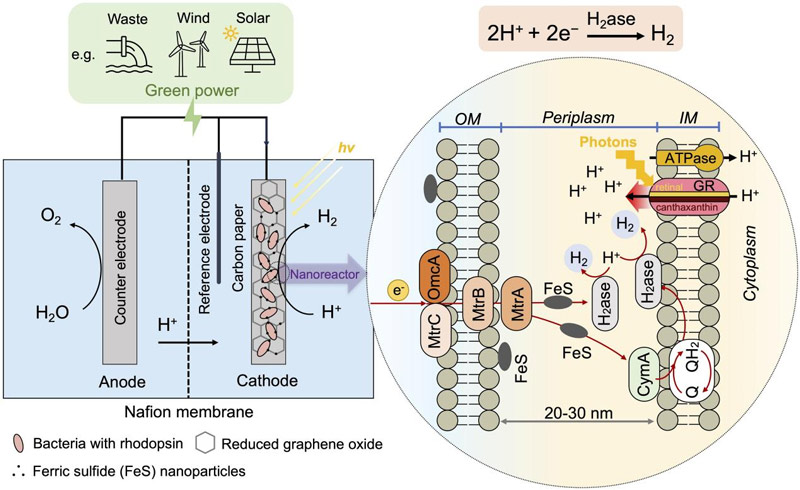
This “bionanoreactor” system has successfully solved the efficiency bottleneck in green hydrogen production through the synergy of several technologies.
Not only does it significantly reduce the energy consumption and cost of hydrogen production, it also provides a safer and more environmentally friendly alternative.
In addition, this modular design concept also has broad potential for the synthesis of other green chemicals, such as formic acid and succinic acid, laying the foundation for the future development of clean energy technology and the green chemical industry.
In addition, the research team proposed that in the future, these engineered cells could printed on carbon fiber cloth to make “artificial leaves” to achieve large-scale hydrogen production.
At the same time, by further optimizing nanomaterials and genetic engineering design, as well as expanding the industrial application of the system, the technology expected to become an important breakthrough in the field of clean energy.
Reference link:
Tu W, Thompson I P, Huang W E. Engineering bionanoreactor in bacteria for efficient hydrogen production[J]. Proceedings of the National Academy of Sciences, 2024, 121(29): e2404958121.