EC3 enzymes actually refer to EC3 enzymes, namely Hydrolase. Here are the details:
Definition and function
Hydrolase is a class of enzymes that use water to catalyze covalent bond cleavage, which can decompose a large molecule into two small molecules and catalyze the hydrolytic cleavage of C-O, C-N, C-C and some other bonds, including phosphoric anhydride bonds.
sort
(1) EC 3.1 acts on ester bonds: Including esterase acting on carboxylate (EC 3.1.1), thioester hydrolase (EC 3.1.2), phosphomonoester hydrolase (phosphatase) (EC 3.1.3), phosphodiester hydrolase (EC 3.1.4), triphosphate monoester hydrolase (EC 3.1.5), sulfatase (EC 3.1.6), diphosphomonomesterase (EC 3.1.7) and phosphotriester hydrolase (EC 3.1.8).
(2), EC 3.2 Glycosyl enzymes: enzymes that hydrolyze O – or S-glycosyl compounds, i.e. glycosides (EC 3.2.1 and EC 3.2.3), and enzymes that hydrolyze n-glycosyl compounds (EC 3.2.2).
(3) EC 3.3 acts on ether bonds: It is divided into enzymes that hydrolyze thioether and trialkyl sulfonium compounds (EC 3.3.1) and enzymes that act on ether (EC 3.3.2).
(4), EC 3.4 acts on peptide bonds (peptidase) : It is also divided into outer peptidase and internal peptidase.
Exopeptidases include aminopeptidase (EC 3.4.11), dipeptidyl peptidase and tripeptidyl peptidase (EC 3.4.14), carboxypeptidase (EC 3.4.16-18), peptidyl dipeptidase (EC 3.4.15), etc.
Endopeptidase can be divided into serine endopeptidase (EC 3.4.21), cysteine endopeptidase (EC 3.4.22), aspartate endopeptidase (EC 3.4.23), metal endopeptidase (EC 3.4.24) and threonine endopeptidase (EC 3.4.25) according to the catalytic mechanism.
(5) EC 3.5 acts on carbon-nitrogen bonds, in addition to peptide bonds: such as urease, can catalyze the hydrolysis of urea to ammonia and carbon dioxide.
(6), EC 3.6 acts on acid anhydride: such as adenosine triphosphatase (ATPase), can hydrolyze ATP to produce ADP and inorganic phosphoric acid, release energy for cell metabolism and other processes.
(7) EC 3.7 acts on carbon-carbon bonds: for example, some lyases can catalyze specific carbon-carbon bond hydrolysis reactions.
(8) EC 3.8 acts on halogen bonds: it can hydrolyze halogen bonds in organic halides.
(9) EC 3.9 acts on phosphorus-nitrogen bonds: such as phosphoamidase.
(10), EC 3.10 acts on sulfur nitrogen bonds: hydrolysis of compounds containing sulfur nitrogen bonds.
(11) EC 3.11 acts on carbon-phosphorus bonds: it can hydrolyze specific carbon-phosphorus bonds.
(12), EC 3.12 acts on sulfur-sulfur bond: catalyzes the hydrolysis reaction of sulfur-sulfur bond.
(13) EC 3.13 acts on carbon-sulfur bonds: hydrolyzes carbon-sulfur bonds.
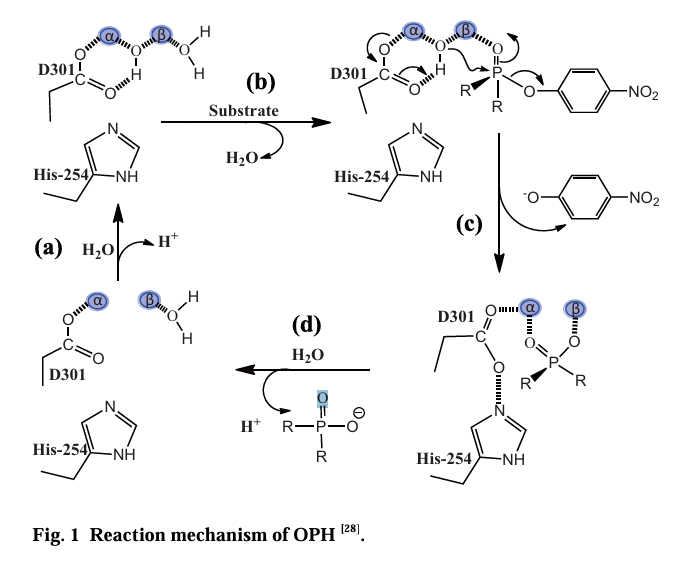
Physiological function
(1) Participate in material metabolism: It plays a key role in the digestion, absorption and metabolism of nutrients such as carbohydrates, proteins and fats.
For example, amylase (EC 3.2.1.1, etc.) hydrolyzes starch, protease hydrolyzes protein, and lipase hydrolyzes fat.
(2) Maintain the stability of the intracellular environment: regulate the concentration and activity of various biomolecules in the cell through hydrolysis, such as the hydrolase in the lysosome can degrade the aging, damaged organelles and biological macromolecules in the cell.
(3) Participate in signal transduction: some hydrolases can hydrolyze signal molecules or their precursors, thereby regulating the activity of signal transduction pathways.
For example, phospholipase C hydrolyzes phosphatidylinositol-4, 5-diphosphate to produce inositol-1,4, 5-triphosphate and diacylglycerol, which are involved in intracellular signal transduction as second messengers.
latest research results of EC3 enzyme:
1. Research progress of ATP synthase
An enzyme electrocatalysis research team led by Zhang Lingling, a researcher at the Tianjin Institute of Industrial Biotechnology, Chinese Academy of Sciences, has made progress in synthesizing ATP from ATP synthases on thylakoid membranes driven by electricity.
In this work, the thylakoid components from the chloroplast grana of spinach were extracted, the grana thylakoid membrane was transformed into a planar thylakoid membrane, and the membrane was loaded on the perfluorosulfonic acid membrane, a proton exchange membrane commonly used in electrochemical ponds, to give the perfluorosulfonic acid membrane additional biological activity.
In this study, the bioactive membrane was assembled in electrolytic water system, and the efficient coupling of ATP synthesis and electrochemical reaction was realized by optimizing the preparation of planar thylakoid membrane and electrolytic conditions.
Under the application of 3V voltage, 8.39±1.37μM ATP is produced within 120min, and the maximum ATP synthesis rate can reach 15nmol cm-2h-1, and the Faraday efficiency is 0.19%1.
Some studies have optimized the application of lipase (EC 3.1.1.3) in biodiesel production.
By modifying lipase through genetic engineering, the activity and stability of lipase under specific reaction conditions are improved, and the production efficiency of biodiesel is significantly improved, while the production cost is reduced.
3. Research on glycosyl hydrolase
In the study of glycosylhydrolase (EC 3.2), scientists have discovered a new type of glycosylhydrolase with unique substrate specificity and catalytic mechanism.
The enzyme can efficiently hydrolyze specific glycosidic bonds, providing a new tool for structural analysis of complex sugars and preparation of bioactive substances.
4. New discovery of peptidase
For peptidase (EC 3.4), a research team has found a specific high expression of endopeptidase in tumor cells, and its activity is closely related to the occurrence and development of tumors.
By inhibiting the activity of this peptidase, the proliferation and metastasis of tumor cells can significantly inhibited, which provides a new potential target for the treatment of tumor.
5. Mining and application of new hydrolase
Through metagenomics technology, a variety of novel EC3 hydrolases have excavated from extreme environments.
These enzymes have unique catalytic properties and the ability to adapt to extreme conditions, and have potential application value in bioremediation, biosensors and other fields.
For example, a hydrolase found in hot springs can maintain high activity under high temperature and high salt conditions and can used to treat specific pollutants in industrial wastewater.
Application cases of EC3 enzymes in synthetic biology:
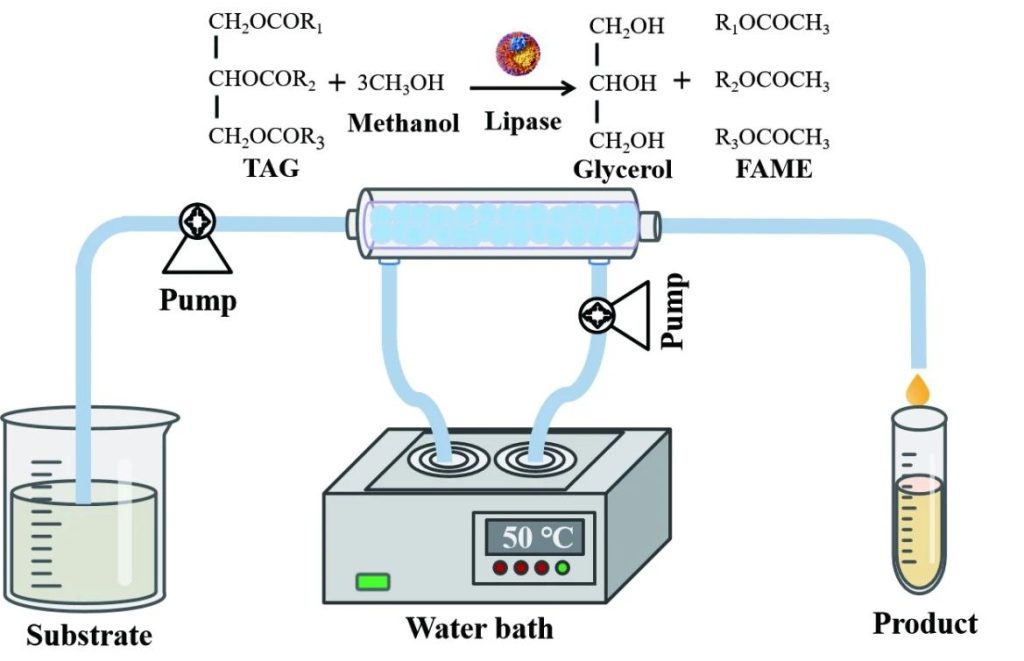
1. Bioenergy field
Production of biodiesel from lipase: Lipase (EC 3.1.1.3) catalyzes the transesterification of fats and alcohols to produce biodiesel and glycerol.
In synthetic biology, lipase genes genetically engineered so that they efficiently expressed in microbial hosts, and then large-scale lipase production performed using microbial fermentation.
The modified microbial cells can used as biocatalysis to achieve efficient and low-cost production of biodiesel, and the reaction conditions are mild and environmentally friendly, reducing the energy consumption and pollution caused by high temperature and high pressure in traditional chemical methods.
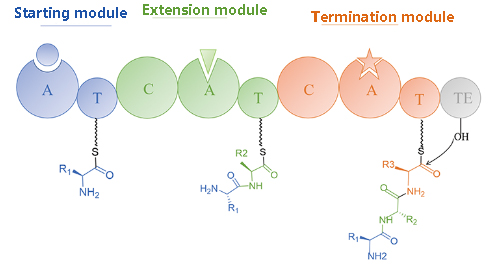
2. Medical field
Synthesis of peptide drugs using peptidases: Peptidases (EC 3.4) can used for the synthesis of peptide drugs.
For example, specific peptidases can modified through directed evolution techniques to recognize and catalyze specific amino acid sequences for condensation reactions, thereby synthesizing polypeptide drugs with specific biological activities.
This method can realize the efficient synthesis of polypeptide drugs, and can accurately regulate the structure and activity of drugs, improve the quality and efficacy of drugs.
Synthesis of drug intermediates using hydrolases: Certain hydrolases can catalyze specific chemical reactions to produce intermediates required for drug synthesis.
For example, in the synthesis of antibiotics, hydrolase used to catalyze the breaking or formation of specific chemical bonds, providing key intermediates for subsequent chemical synthesis steps, thereby simplifying the synthesis route and improving the synthesis efficiency and product quality.
3. Food field
Production of high fructose corn syrup using amylase and glycosylase: In the production of high fructose corn syrup, starch first hydrolyzed to dextrin and oligosaccharides using amylase (EC 3.2.1.1, etc.), which then further hydrolyzed to glucose using glycosylase.
The glucose isomerase (EC 5.3.1.5) then converts part of the glucose into fructose, resulting in high fructose syrup.
The application of these enzymes enables efficient conversion from starch to high fructose corn syrup, providing an important sweetener for the food industry.
Cheese production from rennet: Rennet is an aspartate endopeptidase (EC 3.4.23) that plays a key role in cheese production.
The rennet gene expressed in microorganisms by genetic engineering technology to achieve large-scale production of rennet.
Adding rennet to milk can make milk coagulated accurately, improve the yield and quality of cheese, and can also modified according to needs to meet the needs of different cheese production.
4. Fine chemical industry
Synthesis of chiral compounds by lipase: The synthesis of chiral compounds can achieved by constructing active sites with specific selectivity through protein engineering of lipase.
For example, in the synthesis of some drug molecules, the modified lipase used to catalyze asymmetric esterification or hydrolysis reactions to synthesize drug intermediates or end products with a single chiral configuration, which provides an efficient and green synthesis method for the pharmaceutical industry.
Synthesis of oligosaccharides using glycosylhydrolases: Glycosylhydrolases (EC 3.2) can used for the synthesis of oligosaccharides.
By studying the substrate specificity and catalytic mechanism of glycosylhydrolase, specific monosaccharides or oligosaccharides can linked to synthesize oligosaccharides with specific structure and biological activity by using its reverse reaction or transglycoside reaction.
These oligosaccharides have wide application prospects in food, health care products and medicine.
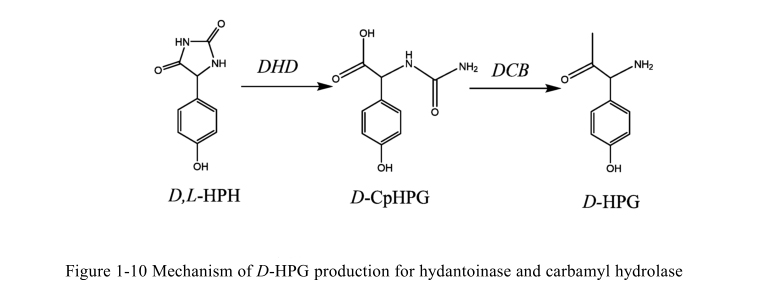
Application of hydrolase in synthesis of drug intermediates
1. Synthesis of D-p-hydroxyphenylglycine by D-carbamoyl hydrolase
D-p-hydroxyphenylglycine is an important intermediate in the synthesis of beta-lactam antibiotics such as ampicillin and cephalosporin.
In the process of enzymatic synthesis, DL-p-hydroxybenzohynine was first hydrolyzed asymmetrically to n-carbamoyl-D-p-hydroxyphenylglycine by d-hydantoylurase. N-carbamoyl-d-amino acid hydrolase then irreversibly converts n-carbamoyl-D-p-hydroxyphenylglycine to D-p-hydroxyphenylglycine.
D-carbamylhydrolase modified by genetic engineering technology, such as random mutation by combining error-prone PCR technology and DNA shuffling technology, and a mutant with increased soluble expression and significantly increased enzyme activity obtained, so that the production efficiency of D-p-hydroxyphenylglycine improved.
2. Synthesis of pregabalin chiral intermediates by nitrile hydrolase
Pregabalin is a gamma-aminobutyric acid analogue that has antiepileptic, anticonvulsant, analgesic, and antianxiety actities1.
In the process of chemoenzymatic synthesis, the racemic isobutylbutyronitrile first selectively hydrolyzed to the chiral intermediate (S) -3-cyano-5-methylcaproic acid under the catalysis of nitrile hydrolase, and then hydrogenated to (S) -3-cyano-5-methylcaproic acid under the action of Ni catalyst. Converted to pregabalin 1.
In addition, in order to improve the catalytic efficiency and reuse performance of nitrile hydrolase, it can also immobilized on the metal-organic framework.
3. Synthesis of chiral drug intermediates by epoxide hydrolase
Epoxide hydrolase is widely used in the synthesis of chiral drug intermediates 23.
It can speed up the conversion efficiency and screening in the biological conversion process to a certain extent, and the reaction is very soft 23.
For example, in the synthesis of some complex chiral drug intermediates, epoxide hydrolases can specifically hydrolyze epoxides to generate hydroxyl compounds with specific chiral configurations, providing key intermediates for subsequent drug synthesis.
4. Alkyd nitrile enzyme synthesis of pulliform drug intermediates
In the synthesis of Pulliform drug intermediates, it found that the alkonitrile enzyme can catalyze the synthesis of chiral silicon-containing structure analogues of pulliform drug intermediates by constructing a variety of alkonitrile enzyme libraries.
At the same time, microorganisms with two enzyme activities of nitrilase and nitrilhydrolase screened, and the whole cell catalysis attempted to prepare hydroxyacetic acid and mandelic acid and other intermediates.
5. Synthesis of D-p-hydroxyphenylglycine by D-heinase
D-hynase plays a key role in the asymmetric hydrolysis of DL-p-hydroxybenzohynase to n-carbamyl-D-p-hydroxyphenylglycine and is an important enzyme in the first step of enzymatic synthesis of D-p-hydroxyphenylglycine.
Its catalytic activity and selectivity directly affect the subsequent reaction and the yield and quality of the final product.