Tripterygium abstract
Obesity is a major health risk, and there is still a lack of effective medication treatment. An effective anti obesity agent, Triptolide, has been found in the roots of Tripterygium wilfordii.
However, an effective synthesis method is needed to better explore its biological utility. Here, we elucidate the 11 missing steps in the biosynthesis pathway of Tripterygium wilfordii glycoside to enable de novo biosynthesis in yeast.
Firstly, we revealed the four oxidation steps catalyzed by cytochrome P450 enzyme, which produce the key intermediate Triptolide.
Subsequently, we demonstrate that non enzymatic decarboxylation triggers the activation of triptolide, leading to a cascade of catechol oxidation driven double bond extension events, resulting in the formation of the characteristic quinone methylated portion of triptolide.
Researchers have developed a method for producing Triptolide from edible sugar.
This work emphasizes the effectiveness of combining plant biochemistry with metabolic engineering and chemistry for the synthesis of complex metabolites.
Main results Tripterygium
1. Functional screening and identification of two types of CYPs in tobacco
Triptolide accumulates in the roots and not synthesized in the leaves. Therefore, researchers searched for CYP genes that were selectively expressed in root tissues and identified 21 candidate genes;
In order to perform functional characterization on selected CYP candidates, Agrobacterium mediated transient expression used in tobacco to combine with the first step characterized genes TwOSC4 and CYP712K1, and combined with each newly selected CYP candidate gene.
Ultra high performance liquid chromatography high-resolution mass spectrometry (UPLC-HRMS) analysis performed on the terpenoid compounds produced in permeated tobacco leaves, and the results showed that two candidate genes, CYP716C52 and CYP81AM1, able to convert pyruvic acid into a series of different compounds.
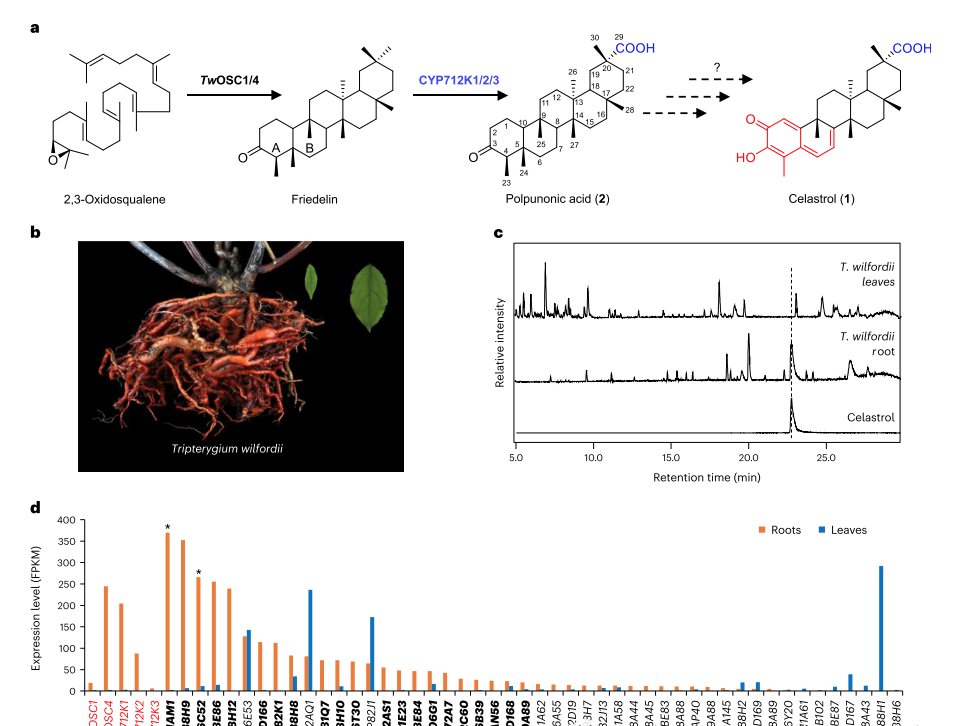
2. CYP716C52 catalyzes the hydroxylation of pyruvic acid at C2 site
Integrating CYP716C52 into the genome of strain AM254-11, which produces pyruvic acid, can avoid the production of glycosylated derivatives into compounds. After isolation and comprehensive analysis of one-dimensional and two-dimensional NMR data from 3L supplemented batch yeast culture, it identified as Wilfornic acid C, indicating that CYP716C52 catalyzes the hydroxylation of Triptolide at C2.
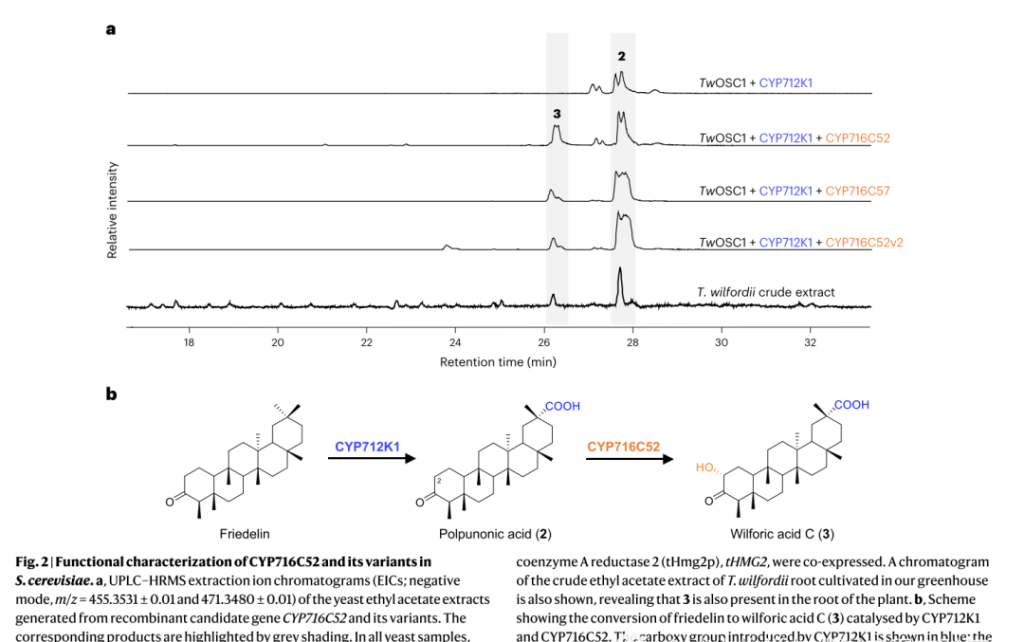
3. CYP81AM1 oxidizes the C24 methyl of pyruvate to carboxyl group
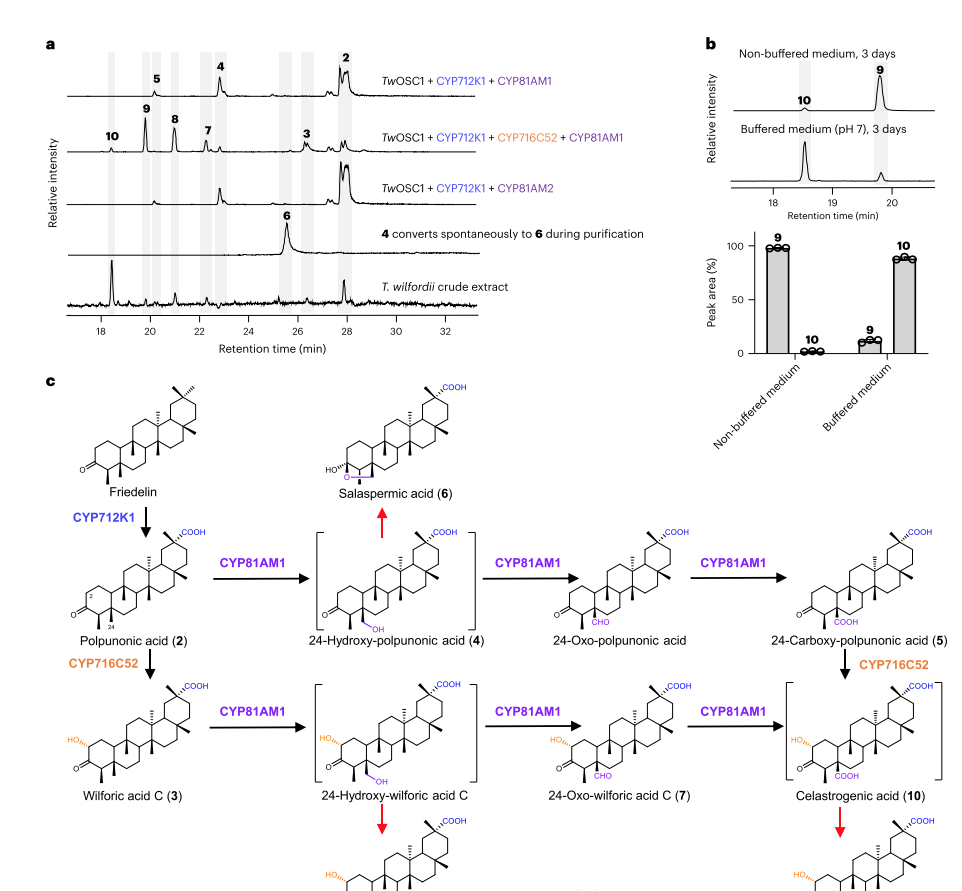
In order to determine the structure of the compounds produced by CYP81AM1, CYP81AM1 integrated into the genome of yeast strain AM254-11, which produces bopyruvic acid. This leads to the conversion of pyruvate into two main products 4 and 5, as previously observed in tobacco. 4 is unstable and converts to the previously reported triterpenoid compound salazic acid. Structural identification of 6 indicates that CYP81AM1 may catalyze the C24 methylation oxidation of pyruvic acid, generating 24 hydroxy pyruvic acid, which then converted to C3 through attack to generate hydroxyl groups.
4. C24 decarboxylation does not require enzymes
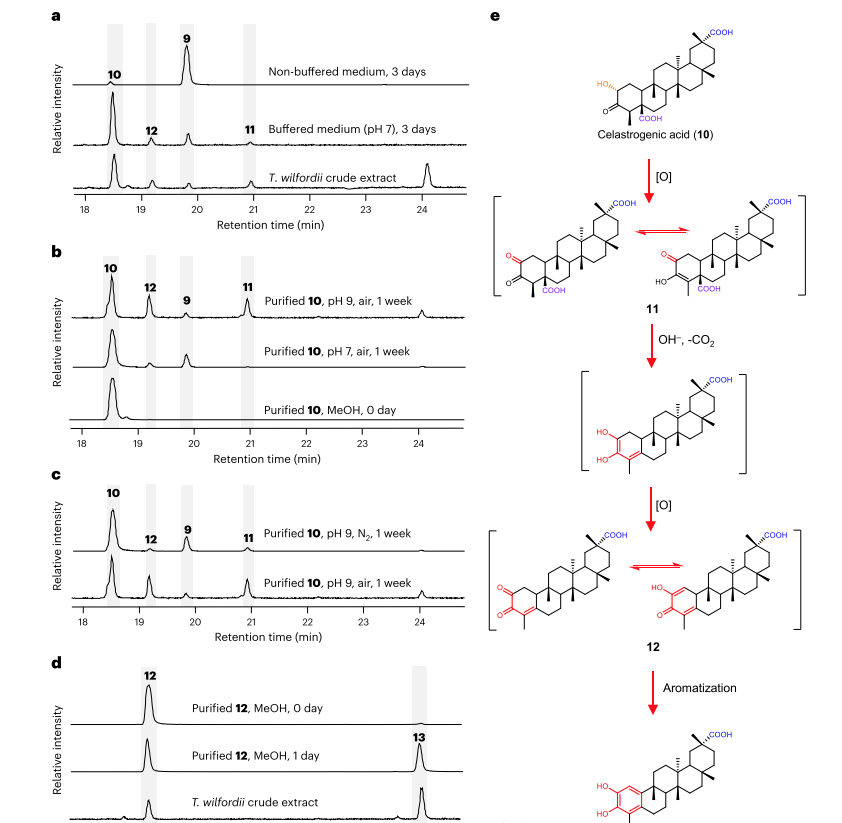
Compared with the structure of Triptolide (10), the structure of Triptolide lacks C24, and one of the next steps must be decarboxylation. There reports of P450 catalyzed decarboxylation reactions, which screened 19 CYPs specifically expressed in root tissues but showed no effect. The decarboxylation of special metabolites can also undergo spontaneous reactions.
Under acidic conditions, the triptolide (10) produced by yeast mainly exists in the form of an ester (9). Yeast cells producing 10 were cultured in neutral pH (pH7) buffered medium, with 10 being the main form and two byproducts 11 and 12. Compounds 11 and 12 are also present in the roots of greenhouse cultivated Thunder God Vine.
These results indicate that 11 and 12 can produced non enzymatic. Increasing the pH value from 7 to 9 will result in a significant conversion of 10 to 11 and 12. O2 plays a crucial role in this reaction in the environment. 12 was isolated from dry plant materials, and its structure cannot determined by nuclear magnetic resonance spectroscopy.
Prove that 12 is unstable. When resuspended in methanol, 12 completely transforms into compound 13, with the same molecular formula as 12 (C29H42O4) (Figure 4d). Subsequently, NMR analysis performed on 13 to confirm its identity as Wilfornic acid A.
5. Cascade reaction of catechol oxidation in series to generate Tripterygium wilfordii glycoside
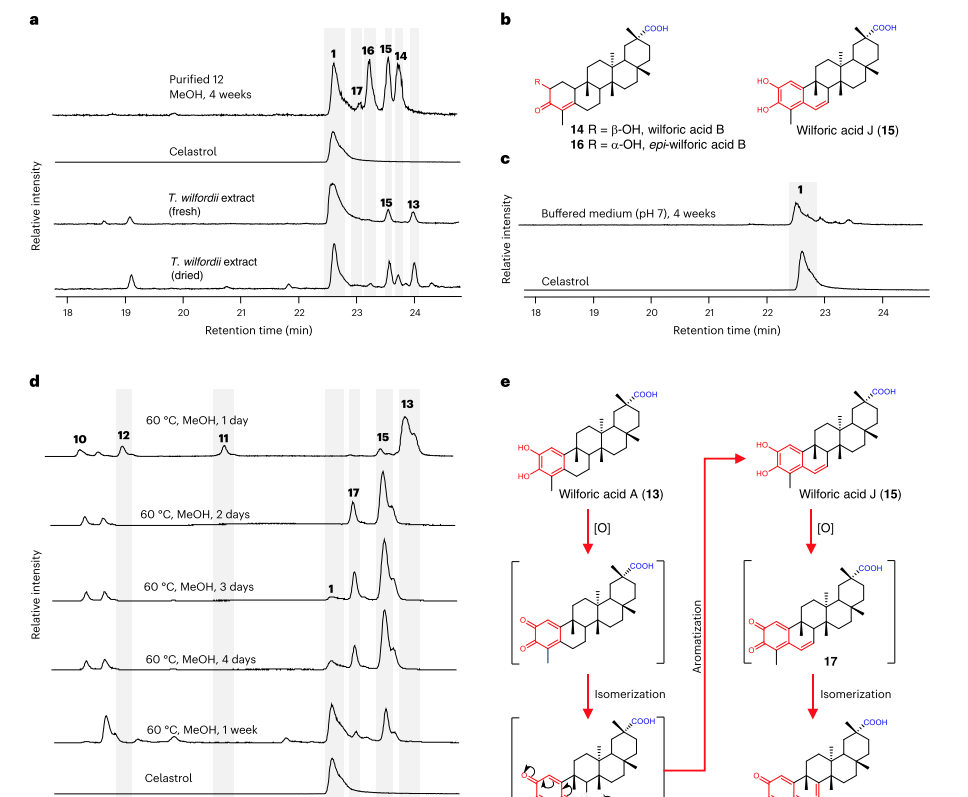
When the methanol solution of 12 exposed to air for 4 weeks, several other compounds observed in addition to 13. Triptolide (1) belongs to these compounds (Figure 5).
The remaining products, compounds 14-17, identified as triterpenoid compounds previously reported, namely Wilforic acid B. Compounds 15 and 16 are a type of triterpenoid compound that has not yet characterized, named Wilfornic acid J and epi Wilfornic acid B, respectively.
To confirm the biosynthetic relationship between these compounds, purified compounds 13-16 exposed to air for 4 weeks and the resulting products analyzed by LC-HRMS. Determine the conversion of isolated Wilforic acid A (13) to Wilforic acid J (15), and Wilforic acid J (15) to Triptolide.
6. Production and semi biosynthesis methods of Triptolide in yeast
After elucidating the steps of the Triptolide pathway, a biosynthetic method for Triptolide in yeast constructed. Firstly, the production strain AM254-15 generated by integrating an additional copy of the cork synthase gene TwOSC1 and six copies of CYP81AM1 into the genome.
Compared with AM254-14, the titers of 9 and 10 increased fivefold (a total of 15.3 mg l-1 for 9 and 10). Cultivate the strain in buffer medium (pH 7) at 30 ° C for 4 weeks and produce Tripterygium wilfordii red pigment through automatic oxidation.
Due to the slow conversion rate of Triptolide (10) to Triptolide (1), which poses a bottleneck in biological production, researchers have established a semi biological synthesis process. The yeast cells first synthesize Triptolide, which then dissolved in methanol and incubated at 60 ℃ for a week to form a semi synthetic route of Triptolide.
Overall, within one week, 10% of 21.4% successfully converted to Tripterygium wilfordii glycoside. Toxic byproducts 14 and 16 did not accumulate significantly.
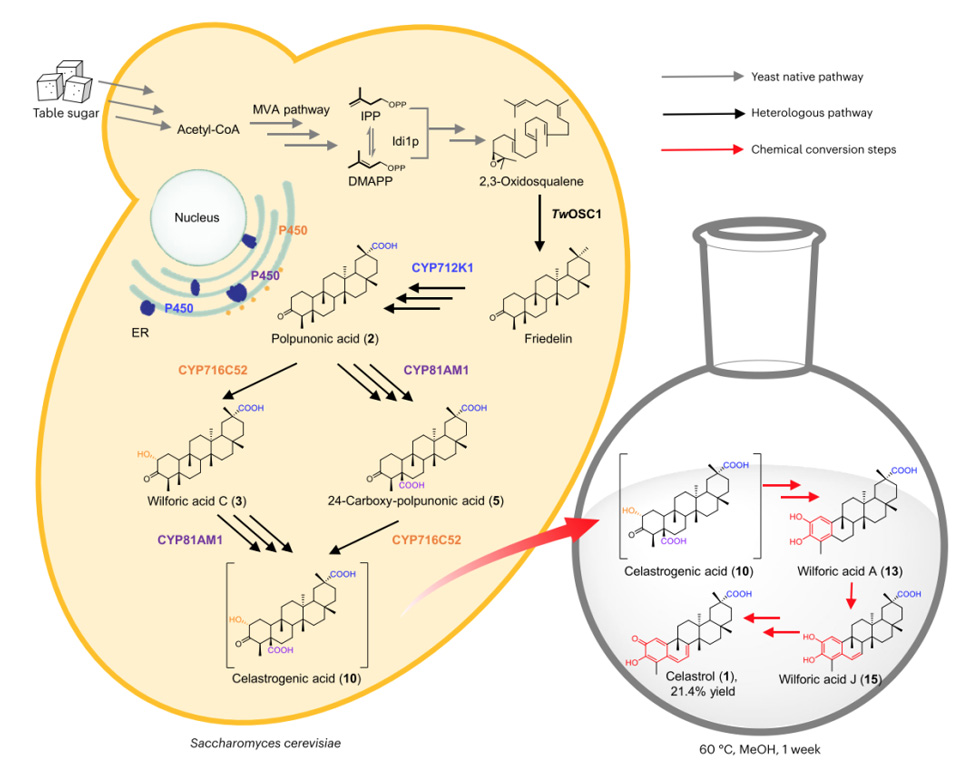
summarize
Researchers applied tissue-specific transcriptome analysis combined with tobacco transient expression screening to characterize the missing steps in the Triptolide pathway. Two potential P450s identified. CYP716C52 catalyzes the C2 hydroxylation of pyruvic acid.
CYP81AM1 oxidizes the C24 methyl group of pyruvate to carboxyl group to produce Triptolide. Triptolide undergoes spontaneous oxidation and decarboxylation steps to remove C24. This decarboxylation triggers a non enzymatic cascade reaction of catechol oxidation driven double bond extension events, resulting in the generation of Tripterygium wilfordii red pigment.
A semi synthetic route of triptolide developed by synthesizing triptolide from yeast cells, which then dissolved in methanol and incubated at 60 ℃ for one week.
Lorem Ipsum is simply dummy text of the printing and typesetting industry. Lorem Ipsum has been the industry’s standard dummy text ever since the 1500s.