Monash University transforms waste into treasure, effectively uses waste carbon sources, and jointly produces bioelectricity and butanol through anode fermentation
With the intensification of global energy crisis and the increasingly serious problem of environmental pollution, how to effectively use waste carbon sources has become a research hotspot in the field of energy and environment.
The extensive use of traditional fossil energy not only leads to a large amount of greenhouse gas emissions, but also causes excessive consumption of non-renewable resources.
The search for sustainable clean energy alternatives is therefore all the more urgent.
Electrofermentation technology, as an important innovation in the field of biotechnology, has attracted more and more attention in recent years.
Compared with the traditional fermentation technology, electrofermentation can dynamically regulate the microbial metabolic process through the guidance of applied electric field to achieve more efficient product generation and energy conversion.
According to the different functions of electrodes, electrofermentation can be divided into anode electrofermentation and cathode electrofermentation, in which anode fermentation uses microorganisms as catalyst, generates current through anodic oxidation of organic substrates, and synthesizes high value-added chemicals.
This approach can not only produce clean energy from waste organic matter, but also provide technical support for the goal of carbon neutrality.
In anode electrofermentation, Shewanella oneidensis and Geobacter reducens are commonly used electroactive strains, which have natural extracellular electron transfer ability.
However, these strains have limited metabolic range and substrate adaptability to efficiently utilize complex or diverse carbon sources.
In response to this limitation, the Monash University research team chose to use Escherichia coli as chassismus to carry out anode electrofermentation research.
E. coli has the following advantages: its genetic engineering toolbox is mature, which can easily realize the construction and regulation of multi-gene pathways;
The metabolic pathways are extensive and can utilize various waste carbon sources;
It has predictable growth performance under both aerobic and anaerobic conditions.
These characteristics make Escherichia coli an ideal candidate for constructing multifunctional engineering strains.
By introducing the MtrCAB-CymA electron transfer pathway of Shiwanella, the team endows Escherichia coli with electroactivity, and integrates the synthetic pathway of isobutanol and 3-methylbutanol, and realizes the synchronous production of bioelectricity and advanced fuel through anode fermentation.
This research has demonstrated the potential of anode electrofermentation, which not only expands the application range of Escherichia coli, but also provides a new idea for the high-value utilization of waste carbon sources.
The work was published in Green Chemistry, Entitled “Co-production of bioelectricity and butanol by engineered Escherichia coli fed organic wastes in anodic. fermentation “.
Through genetic engineering, the research team introduced the MtrCAB-CymA pathway of Shewanella into Escherichia coli to give it electrical activity.
The MtrCAB-CymA pathway is able to transfer electrons inside and outside the cell membrane, thus enabling electron exchange between the cell and the electrode.
To achieve fuel synthesis, the alsS-kivd-adhA gene cluster was also integrated.
The alsS gene catalyzes the production of pyruvate into glycolactone, while the kivd and adhA genes convert the intermediate metabolites into isobutanol and 3-methylbutanol.
The engineered strains cultured under aerobic conditions successfully produced isobutanol (185 mg /L) and 3-methylbutanol (47 mg /L), which verified the function of the synthetic pathway.
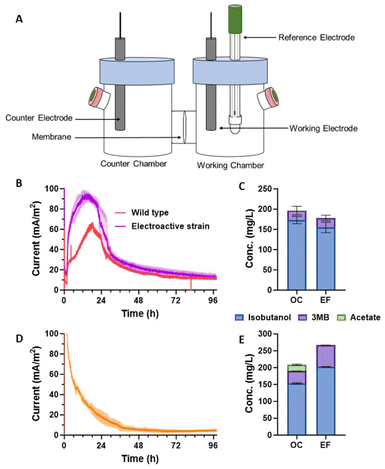
The modified Escherichia coli showed good performance in the electrofermentation system.
First, the current output and fuel synthesis ability of the modified Escherichia coli evaluated when glucose used as carbon source.
Under the condition of electrode connection, the maximum current density produced by the system was 95.2±2.7 mA/ m², and the peak appeared after 14.5 hours of culture.
At the same time, the yield of isobutanol was comparable to that of the open-circuit system, indicating that electron transfer to the electrode did not significantly interfere with the fuel synthesis.
In addition, the electron transfer of the electrofermentation system reached 0.1 mmol (within 96 hours), showing significant electrochemical activity.
The study further evaluated the performance of different waste carbon sources in electrofermentation systems, including glucose, glycerol and acetic acid.
The experimental results showed that glycerol had the best performance as a carbon source, which not only produced the highest isobutanol yield (232 mg /L), but also achieved 0.14±0.02 mmol electron transfer, which was significantly higher than glucose and acetic acid.
The change of glycerol concentration has obvious effect on the performance of the system.
Excessive glycerol concentration may lead to intracellular REDOX imbalance, produce more acetic acid byproducts, and reduce the efficiency of electron transfer to the electrode.
25 mM glycerin is the optimal concentration.
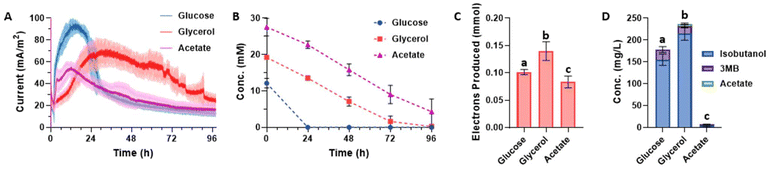
To investigate the effect of electrode surface area on electron transfer efficiency and isobutanol yield, the team increased the electrode surface area from 8 cm² to 40 cm².
The experimental results showed that the larger electrode surface area significantly increased the amount of electron transfer, from 0.14±0.02 mmol to 0.59±0.03 mmol within 96 hours, an increase of 420%.
In addition, the larger electrode surface area significantly improves the REDOX balance, reduces the by-product generation of acetic acid, and shows higher electron transfer efficiency.
Although the electron transfer efficiency significantly improved, the isobutanol yield did not change significantly with the increase of electrode surface area.
Isobutanol yield at 40 cm² electrode was slightly lower than that at 8 cm² electrode (228 mg/ L and 232 mg/ L, respectively) (p = 0.48).
This suggests that isobutanol synthesis may limited by substrate availability and metabolic flux rather than by electron transfer efficiency.
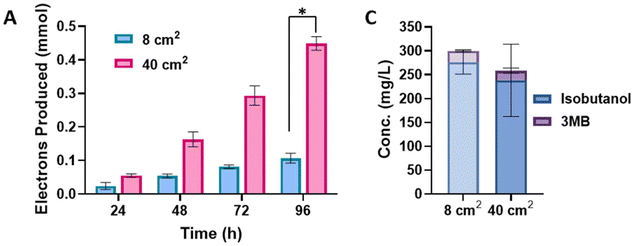
In this study, for the first time, the goal of producing bioelectricity and advanced biofuels simultaneously with engineered Escherichia coli in an electric fermentation system realized.
By introducing the MtrCAB-CymA pathway to the strain, and integrating the ALS-KIVD-ADHA gene cluster to construct isobutanol and 3-methylbutanol synthesis pathway, the modified strain showed excellent electron transfer ability and fuel synthesis efficiency under anaerobic conditions.
Glycerol found to perform best as a waste carbon source, not only producing the highest isobutanol yield (232 mg /L), but also achieving the highest electron transfer (0.14±0.02 mmol).
At the same time, increasing the electrode surface area to 40 cm² significantly improves the electron transfer efficiency and reduces the REDOX imbalance, showing important potential for optimizing the electrofermentation system.
In summary, this study not only provides a new idea for the efficient resource utilization of waste carbon sources, but also shows the broad application prospect of engineered microorganisms in the field of clean energy.
In the future, through system optimization and industrialization practice, electric fermentation technology is expected to become an important means of sustainable production of energy and chemicals.
Reference link:
Jong B, Haritos V S. Co-production of bioelectricity and butanol by engineered Escherichia coli fed organic wastes in anodic fermentation[J]. Green Chemistry, 2024.